Technical Content: High
Read Time: 15 minutes
With the rapid advancement of computer technology, full numerical modelling is now routinely carried out in geotechnical engineering. Producing a full numerical model is not straightforward; if the numerical analysis is not applied properly, the output can lead to defective design and ensuing failure. However, if used with skill and care, full numerical modelling has enormous potential to explain the engineering behaviour of geotechnical structures and produce optimal and safe design.
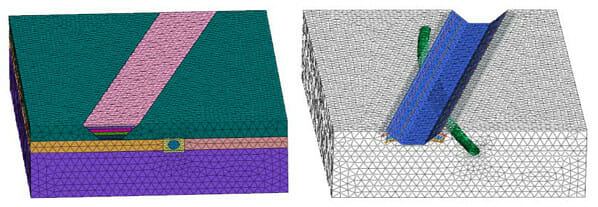
In Part 1 of this article I introduced the technique, and described both its advantages and disadvantages. In Part 2, I described two case studies to show how the technique can be applied to forensic investigation of geotechnical and other soil/structure failures.
In this final part, I will describe two more cases studies, exemplifying the power of the full numerical approach.
CASE STUDY 3: TIEBACK WALL FAILURE IN SOUTH KOREA [2]
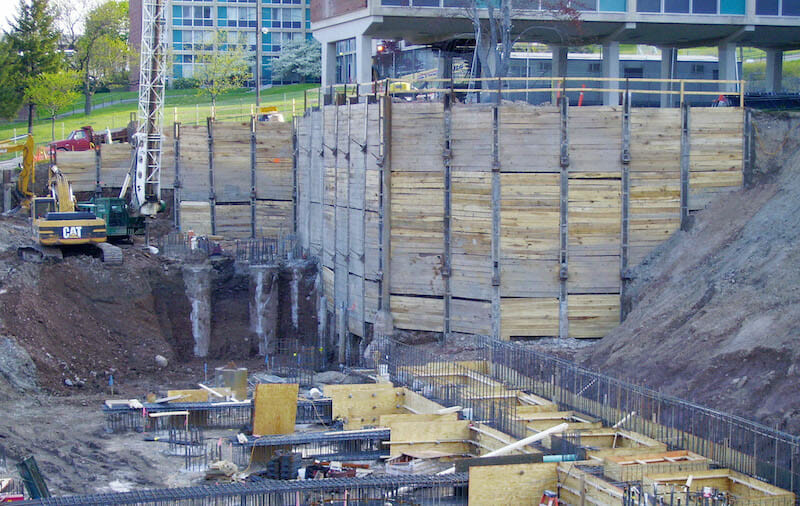
The retaining wall consisted of an 18.3m high, cast-in place pile wall, supported by 9 layers of ground anchors, plus a further 21.7m high H-pile wall supported by numerous layers of rock bolts, with a 2m toe embedment, to retain a total height of 38m.
Based on the site investigation, a fracture zone was noted in the hard rock layer at depths below 30.4m.Sequential excavation proceeded until a catastrophic wall failure occurred after the final excavation stage.
After the collapse, a geotechnical expert performed a full numerical analysis to model the sequential behaviour and subsequent failure.
The response of the anchored retaining wall was simulated by two-dimensional, non-linear finite element analysis. The shear strength reduction method was used to predict the potential failure planes in the anchored wall structures. With the input of sequential excavation stages, the model successfully simulated the progression of the failure plane and captured the change in failure mechanism.
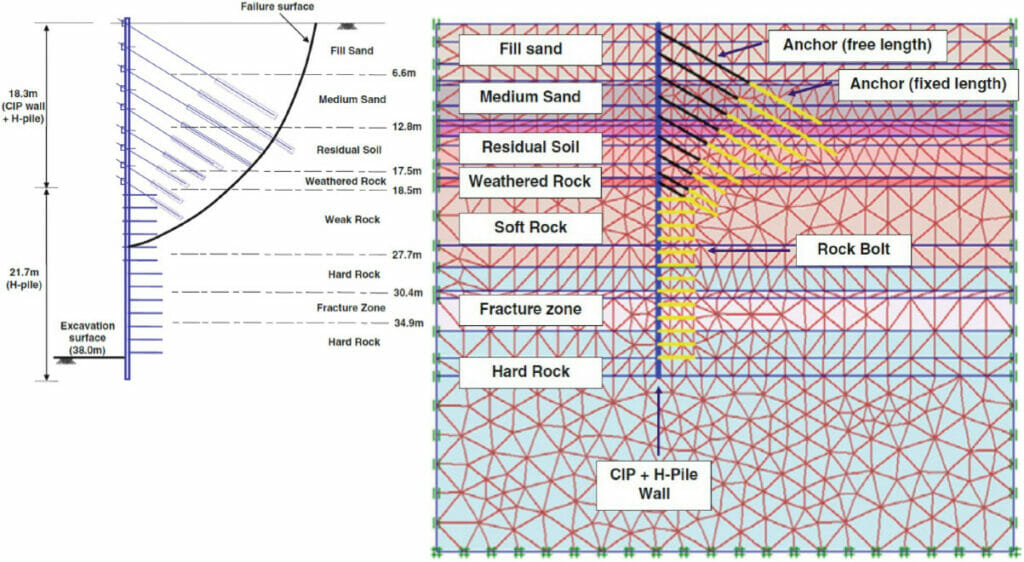
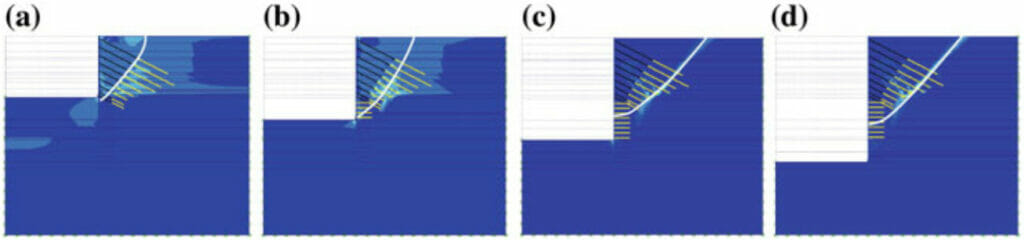
The predicted failure surface shape was a good representation of the real incident failure surface, showing that the numerical method accurately modelled the real life incident. The model confirmed the failure mechanism and failure to take the fracture zone into account. If the model had been used as part of the design, the incident could have been predicted and avoided.
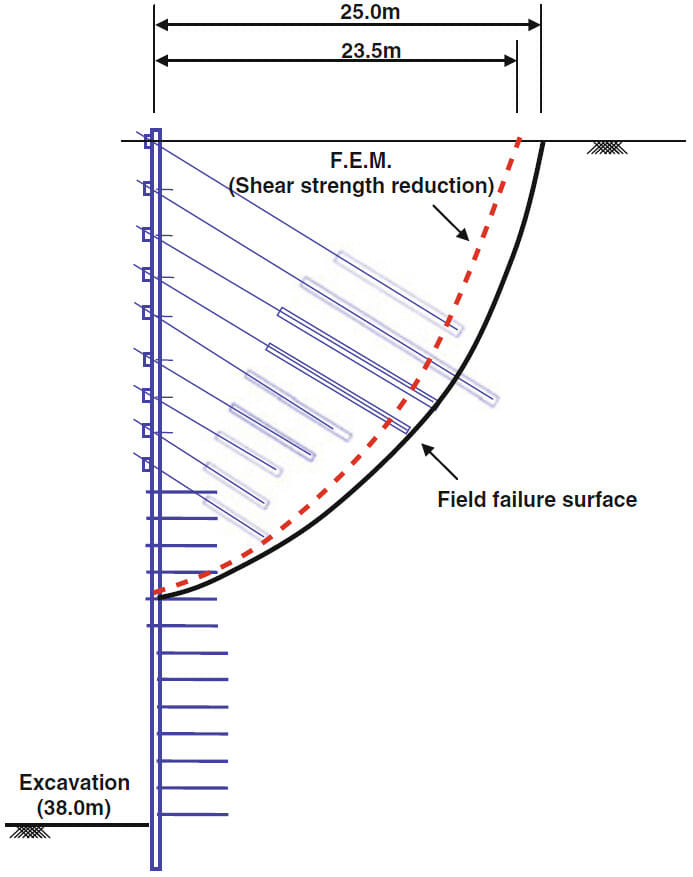
CASE STUDY 4: BUILDING-OVERTURNING IN SHANGHAI, CHINA [4]
On 27 June 2009, a 13-storey building within a construction site (labelled in the images below as Building 7) abruptly fell over with little warning, killing one worker. Prior to the incident, a 4.6 m deep basement excavation was ongoing on the south side of both Building 6 and Building 7. The excavated soil was dumped on the north side of the two buildings and formed 10m high Stockpile 1. As recorded by the local weather station, about half an hour before the building toppled, there was an intense rainfall event lasting for 5 hours.
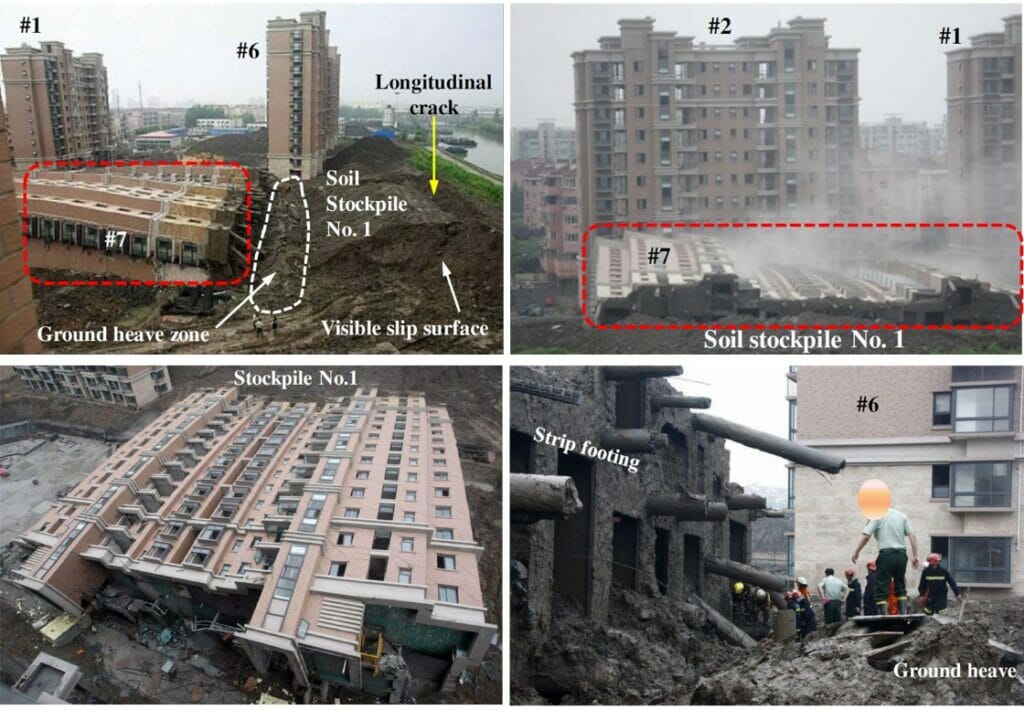
Top Right: right after toppling of Building 7
Bottom Left: view of Building 7 after failure
Bottom Right: view of the foundation and ground below
The preliminary conclusion from the Forensic Diagnosis Committee was that horizontal ground movement, caused by unequal lateral earth pressures on the two sides of Building 7, sheared off the underlying piles, causing the building to topple to the ground.
Considering the complexity of this failure, a three-dimensional full numerical analysis was conducted to verify the postulated failure mechanism.
The three-dimensional model included Building 6, Building 7, Stockpile 1 and the 4.6m deep excavation. The building superstructures, pile foundations and temporary earth retaining structures of the excavation were all modelled as per the actual site conditions. The simulated construction procedures duplicated the following on-site activities:
(1)Construction of Building 6 and Building 7
(2)Formation of Phase 1 of Stockpile 1 (4 m high) behind the buildings
(3)Consolidation of subgrade underneath the stockpile for six months
(4)Excavation of the 4.6 m deep basement and formation of Phase 2 of Stockpile 1 (10 m high)
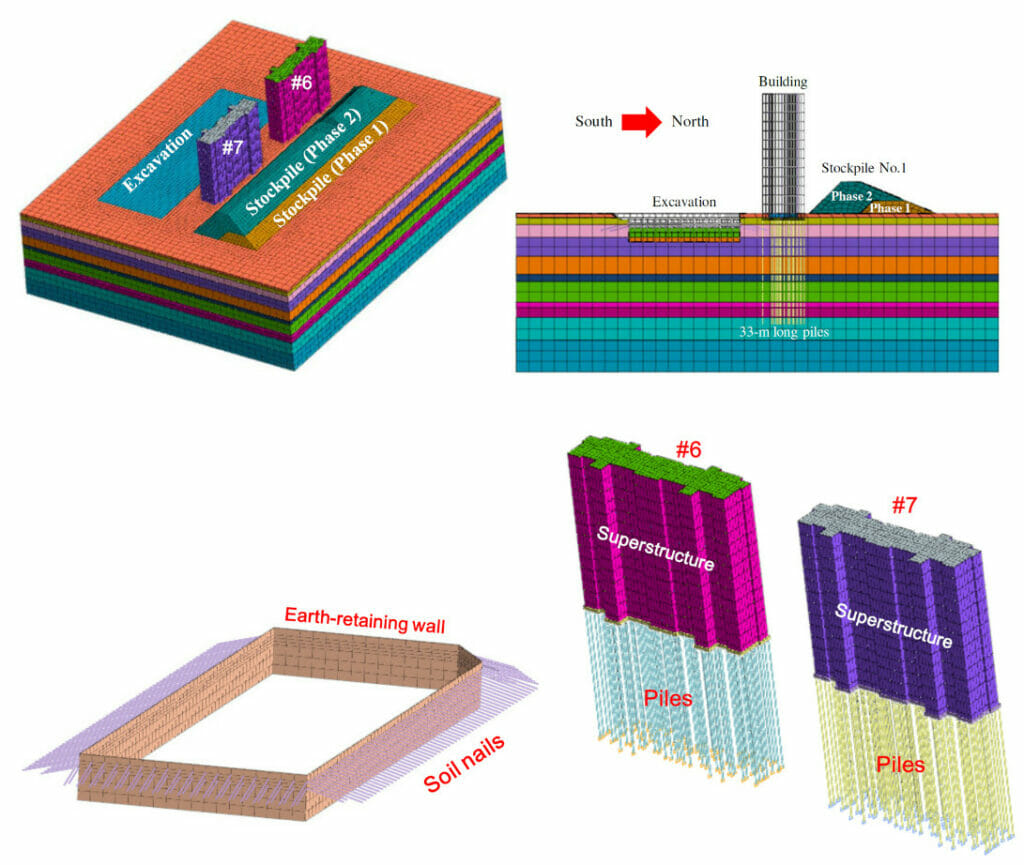
Top Right: Side View
Bottom Left: Temporary Earth Retaining Structures for the excavation
Bottom Right: Superstructures and pile foundations of the buildings
Surprisingly, the simulated building displacements at the completion of excavation and stockpiling indicated a slight northward (instead of southward) inclination of the two buildings. The simulated northward inclinations of about 0.091% for Building 6 and 0.10% for Building 7 were unnoticeable. Moreover, the predicted maximum structural forces of the underlying piles were well within their design capacities and Stockpile 1 was still in a stable state.
These simulation results were consistent with the following two facts:
(1) No obvious sign of inclination was reported for either building before the abrupt failure
(2) The post-failure examination on the piles left in place showed that they had undergone either compressive or tensile failure, not shearing or bending failure, as preliminarily concluded by the Forensic Diagnosis Committee.
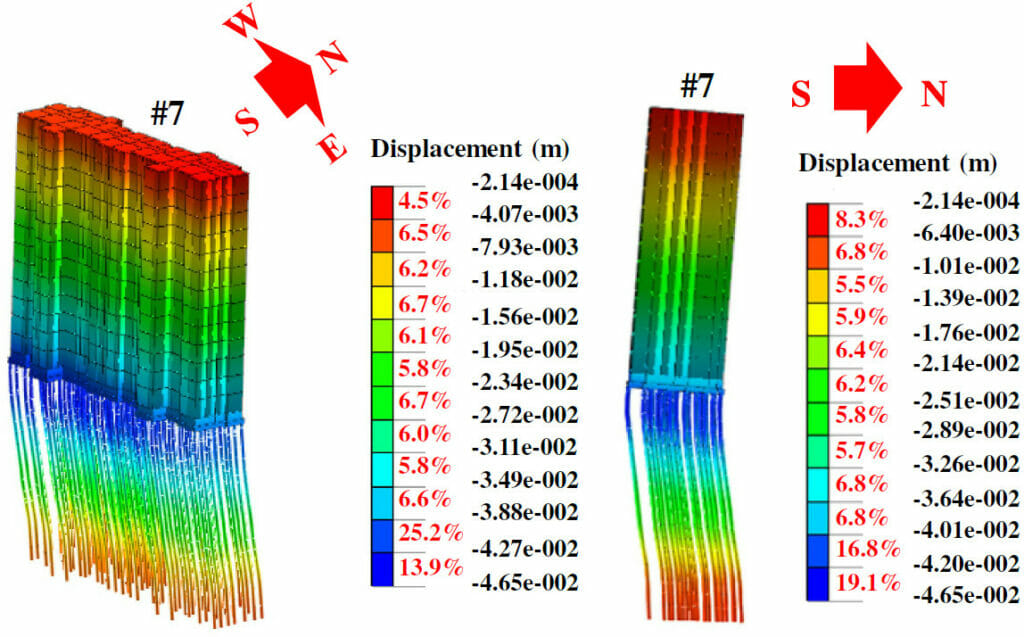
To further investigate the true causation and failure mechanism, the heavy rainfall event was simulated in the model by saturating the stockpile. Stockpile 1 underwent a base failure with significant ground heave, extending below the footing of Building 7, as was actually observed. The simulated stockpile behind Building 6 was still in a safe condition, evidenced by no substantial ground heave below Building 6. This analysis demonstrated that the intense rainfall was a decisive factor, which triggered a deep-seated slip failure of the stockpile and exerted an upward load underneath the north side of Building 7. Consequently, the building fell over suddenly in the southward direction.
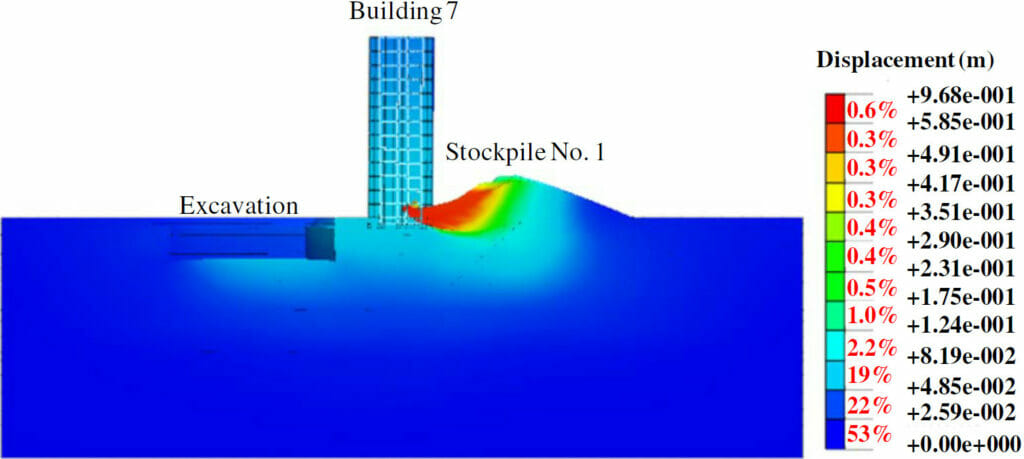
Left: side view of simulated total ground displacement
Right: top view of simulated vertical ground displacements (positive, heave; negative, settlement)
CONCLUSION
The four case studies presented above (and in Part 2) only illustrate a few of the capabilities of full numerical analysis, and how it can assist with forensic investigation. If the analysis is performed properly, it can be applied successfully to various other types of cases as well.
With periodic updates to the analysis software, the process has become so user-friendly that even graduate engineers can perform the analyses with little guidance. This, however, might lead to the danger of misunderstanding either the way the software works or its limitations, as well as potentially using the wrong inputs or misinterpreting the results (i.e. the ‘rubbish in – rubbish out’ issue that effects all software).
Geotechnical engineering is part art, part science. The results of software analyses should be used to provide important evidence for judgments to be based on, and ‘well-winnowed’ experience should prevail.
ABOUT THE AUTHOR
Er. Zhuang Shimin is a Professional Engineer with a background in the design and construction of large-scale geotechnical solutions in Singapore and Hong Kong, including deep basements, retaining walls, reinforced soils, pipe jacking, deep shafts, deep foundations, pile-enhanced raft, segmental and cut & cover tunnels. He is experienced in both 2D & 3D geotechnical finite element analysis.
[1] https://www.midasgeotech.com/blog/influence-of-exc…
[2] S.S. Jeong, Y.H. Kim and M.M. Kim (2016) Developments in Geotechnical Engineering: Forensic Geotechnical Engineering, Chapter 27 Failure Case Study of Tieback Wall in Urban Area, Korea, Springer, India (ISBN: 978-81-322-2376-4)
[3] https://pilebuck.com/foundation/soldier-pile-laggi…
[4] Tan, Y., W. Jiang, H. Rui, Y. Lu, and D. Wang. 2020. “Forensic Geotechnical Analyses on the 2009 Building-Overturning Accident in Shanghai, China: Beyond Common Recognitions” J. Geotech. Geoenviron. Eng. 146 (7): https://doi-org/10.1061/(ASCE)GT.1943-5606.0002264